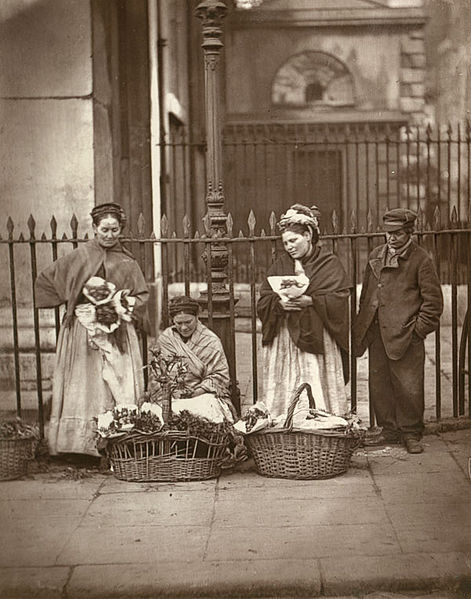
Figure 1 – John Thompson, Flower Girls in Front of Concvent Garden, 1877. From the Wikicommons and in the public domain.
John Thomson (1837 – 1921) was one of the great Scottish photographers of the nineteenth and early twentieth centuries. In his early career he gained notariety as a travel photographer and was one of the first photographers to document the far east. In his later career he became a society photographer in Mayfair and was awarded the royal warrant.But what he is most remembered for today is his partnership with radical journalist Adolphe Smith. The two collaborated in the production of a monthly, Street Life in London, between 1876 – 1877, which was subsequently published in book form in 1878. The project was to document London street life, the lives of the people who scratched out there living invisible and just beneath the eyes of the middle and upper classes. These were images of chimney sweeps, and flower girls, and peddlars.
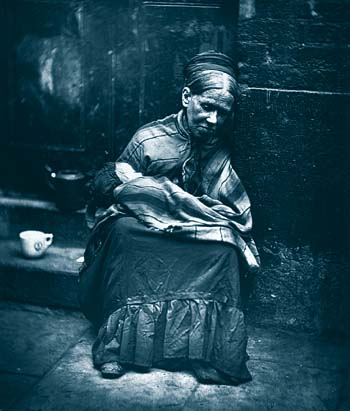
Figure 2 – John Thompson, The Crawlers, 1876-1877, From the Wikipedia posted by catus.man and in the public domain.
These powerful images represent one of the first great social commentary photographic series. and served to create a visual resonance to the stories of Charles Dickens. Life was not rosey on the streets of London. Take, for instance, figure 1, which shows flower “girls” in front of Convent Garden. They are not Eliza Doolittle. In Smith and Thompson’s own words:
“When death takes one of the group away, a child has generally been reared to follow in her parents’ footsteps; and the” beat” in front of the church is not merely the property of its present owners, it has been inherited from previous generations of flower-women. Now and then a stranger makes her appearance, probably during the most profitable season, but as a rule the same women may be seen standing on the spot from year’ s end to year’s end, and the personages of the photograph are well known to nearly all who are connected with the market…”
Truly, in creating Street Life in London, John Thompson defined the profession of photojournalism. The powerful images that chronicle social injustice in the twentieth and twenty-first centuries have their roots, and owe their essence to these photographs by John Thompson.