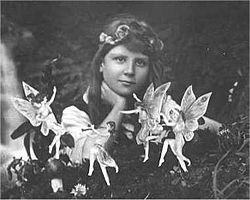
Figure 1 – The first of the five Cottingly Fairies photographs, 1917, from the Wikicommons and in the public domain in the US.
Well, here I am fresh from the season finale of “Downton Abbey,” and my thoughts are with the early twentieth century English countryside. So what better story is there than the great photographic “fake before Photoshop” known as the “Cottingley Fairies.” It is a wonderful story about how two young girls duped there elders for over sixty years.
In 1917 ten year old Frances Griffiths was staying with her cousin sixteen year old Elsie Wright in the village of Cottingley in West Yorkshire. There were stories that fairies lived beside the stream or “beck,” which was at the bottom of the garden. Frances and Elsie borrowed Elsie’s father’s camera and went to prove the existence of the fairies by photographing them.
The girls produced two photographs in 1917 of themselves with the fairies. Elsie’s father thought them pranks, but his wife was convinced that they were real. The story took off, when in 1919 Elsie’s mother attended a meeting of the Theosophical Society about “Fairy Life” and she showed the photographs to the speaker, Polly Wright. They were subsequently displayed at the annual meeting of the society and came to the attention of Edward Gardner, who was a leading member of the society. Gardner had the negatives examined by photography expert Harold Snelling, who declared them genuine. Perhaps, needless-to-say, Snelling supplied prints for sale at Gardner’s lectures.
The story might have rested there, were it not for the fact that they came to the attention of Sherlock Holmes author and spiritualist Sir Arthur Conan Doyle, who was writing an article on fairies for the Christmas Issue of “The Strand.” Doyle was initially skeptical and had the photographs examined by experts at Kodak, who again declared them to show no signs of fakery, but pointed out that they could not certify that they were authentic images of fairies. Experts from Ilford , on the other hand saw definite signs of faking. The photographs were published along with Doyle’s article.
In 1920, Doyle contracted Gardner to confirm or disprove the story of the photographs. In Gardner’s words:
“I went off, to Cottingley again, taking the two cameras and plates from London, and met the family and explained to the two girls the simple working of the cameras, giving one each to keep. The cameras were loaded, and my final advice was that they need go up to the glen only on fine days as they had been accustomed to do before and tice the fairies, as they called their way of attracting them, and see what they could get. I suggested only the most obvious and easy precautions about lighting and distance, for I knew it was essential they should feel free and unhampered and have no burden of responsibility. If nothing came of it all, I told them, they were not to mind a bit.”
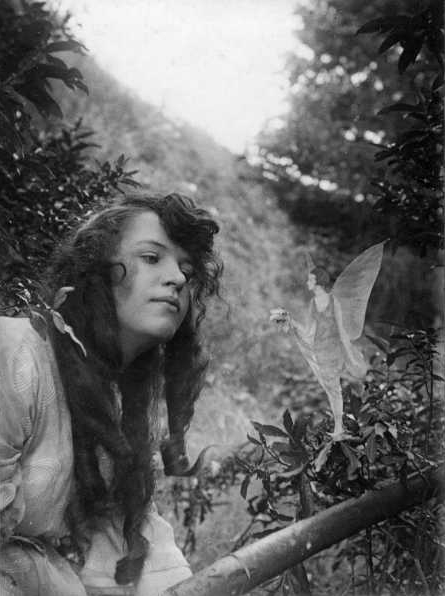
Figure 4 – The fourth photograph of the Cottingley fairies, Fairy Offering Posy of Harebells to Elsie, 1920. From the Wikicommons and in the public domain in the US.
Of course, the girls insisted that the fairies would not show themselves in the presence of anyone else, and here’s a surprise, they captured three more fairy pictures (or is it pixie pics?). Thus, began the long and controversial saga of the Cottingley Fairies.
It was not until 1983 that the cousins admitted in an article published in the magazine “The Unexplained” that the photographs had been faked, although both maintained that they really had seen fairies. Elsie had copied illustrations of fairies from a popular children’s book of the time, “Princess Mary’s Gift Book (1914).” They said they had then cut out the cardboard figures and supported them with hatpins, disposing of their props in the beck once the photograph had been taken. It was an amazing prank, perpetrated on the gullible for sixty-six years. Frances died in 1986, and Elsie in 1988.
If we look at the pictures today, we are surprised by their crudity and the fact that they could fool people for so long. It is not so much a study in photographic fakery as it is a lesson in wishful thinking. Who would not hope that Tinkerbell is real? The remaining question is what would Lord Grantham, Lady Cora, and Lady Mary think?